A Brief Introduction
The field of cosmology attempts to answer very big questions about our universe: What were the earliest moments of the universe like? How did the early universe evolve into the large scale structure we observe today and how will it continue to evolve in the future?
In the universe today, we observe galaxy clusters and superclusters, millions of light-years across, containing massive amounts of matter in the form of planets, black holes, neutron stars, brown dwarfs, and other compact objects. However, in its earliest stages, soon after the Big Bang, the universe was a hot, dense plasma of photons, electrons, and baryons (namely protons and neutrons). These particles were constantly interacting at tremendously high energies.
The early universe and the CMB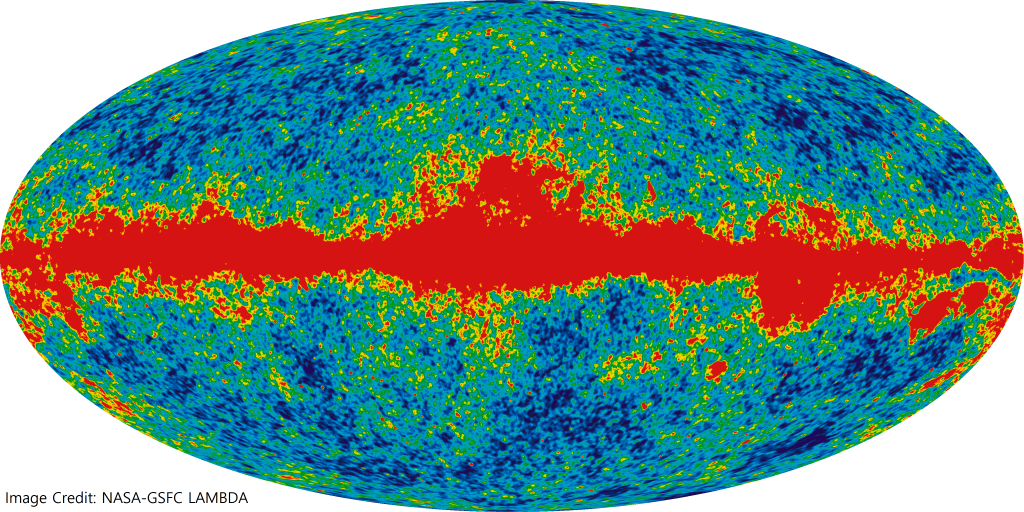
After the big bang, the universe is filled with fundamental particles like quarks, electrons, photons and neutrinos. Then, protons and neutrons are formed. As the universe was expanding and the temperature is cooling, protons and neutrons started to form nuclei. In this phase, the universe’s temperature was still high and therefore the universe is opaque. After the universe got to the point where the matter and energy in the universe is equal, the universe became matter dominated, but most of the matter is ions. Later, the number density of ions in the universe became equal to the number density of neutral atoms. That is defined as the epoch of recombination. After the baryonic component of the universe change from being ionized to being neutral, the universe came to the epoch of photon decoupling. This is the time when the rate at which photons scatter from electrons became smaller than the Hubble parameter which is the used to describe the expansion rate of the universe. This means photons are unlikely to interact with electrons. As a result of photon decoupling, photon went through their last scattering from an electron. These photons are CMB photons and they form the last scattering surface. These CMB photons have been streaming from the last scattering surface freely without interaction with other electrons.
In today’s universe, most of the light comes from the Cosmic Microwave Background instead of stars and CMB is uniform and at a temperature around 2.725 Kelvin. Although there is such large amount of CMB photons, they have not been discovered until 1964. Robert Wilson and Arno Penzias found the Cosmic Microwave Background by accident. They were radio astronomers working at Bell Laboratories. They found a constant “noise” from the sky and they tried everything to reduce “noise” in their system. The explanation was finally provided by Robert Dicke and his research team in Princeton University. They predicted that there should be radiation relic from an early, opaque, dense and hot state of the universe and the discovery from Wilson and Penzias confirmed this prediction. The CMB can tell us a lot about the sate of the universe at the time of last scattering and it is an essential evidence for the Big Bang theory
The 21cm Emission Line and the Current Universe
About 90% of the interstellar medium is in the form of neutral and ionized hydrogen gas. Emission of photons at the 21 cm wavelength occurs when hydrogen atoms undergo a transition at the ground state. The energy of these atoms is slightly higher when the spins of the electron and the proton are aligned than when they are opposite. Therefore, if the electron has spin in the opposite direction to the proton, it will eventually change spin direction, releasing energy in the form of a 21 cm wavelength photon.
Due to the expansion of the universe, light redshifts, or increases in wavelength, as it travels. If some light is known to have been emitted as 21cm radiation but is observed with a longer wavelength, the distance between the source of emission and Earth can be inferred from the amount of redshift. In this way, astronomers can make three dimensional maps of neutral hydrogen throughout large volumes of the universe. These maps can be used to further our understanding of the “dark ages” of the universe, and to investigate dark energy via measurements of the universe’s expansion.
By observing the universe at different redshifts, we can look back in time. The EXperiment for Cryogenic Large-Aperture Intensity Mapping (EXCLAIM) telescope hopes to do just that. Once the telescope launches in 2024, EXCLAIM will observe bright spectral lines emitted by singly-ionized carbon [CII] and carbon monoxide (CO) from the present up to redshift z = 3.5. Because [CII] and CO are good tracers for star formation activity, this information will help us understand why the star formation rate in galaxies peaked several billion years ago, corresponding to a redshift z=2, and slowing down since then.
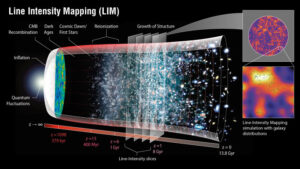
EXCLAIM uses a novel technique called line-intensity mapping that measures the integrated surface brightness rather than flux. Line intensity mapping observes the integral surface brightness of redshifted line emission from galaxies over a broad range of redshifted wavelengths simultaneously, making it ideal for studying evolution.